Our Research Toolsets

Our Research Interests
Electrifying and decarbonizing key industrial sectors, including chemical and materials industries, as well as transportation and aviation, is a pressing mission of our time. Industrial players and governments worldwide have set ambitious targets to reduce carbon emissions, which need to be decreased to net zero around mid-century in order to mitigate global warming and climate change. For such deep decarbonization, it is crucial to build green technologies powered by materials that can electrify key chemical transformations at a capacity of over megawatts and maintain performance throughout thousands of hours of operation. However, there is no time left for materials discovery as usual, as inventing new materials is a painstakingly slow process, while the need for green technologies is urgent.
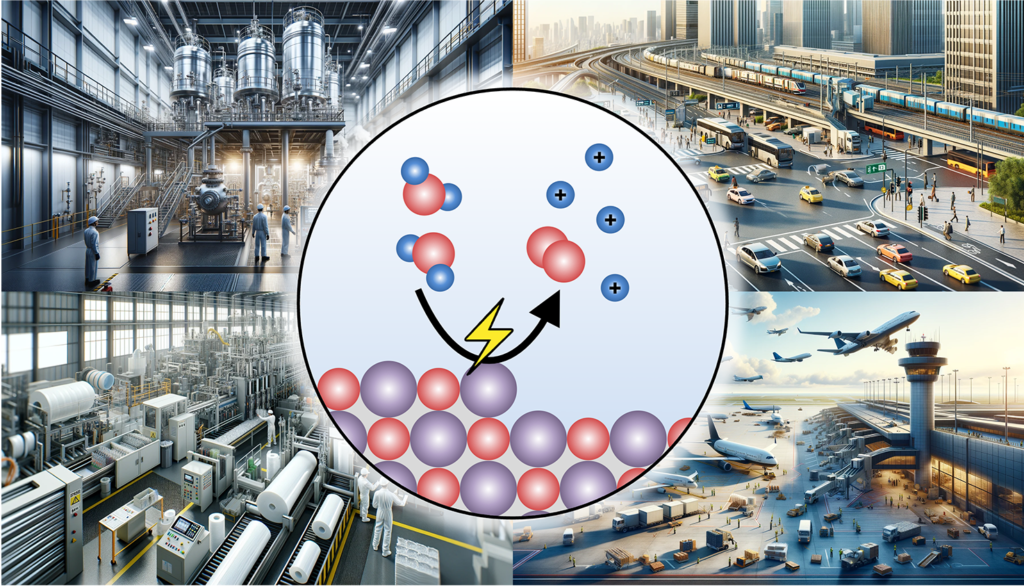
Understanding and optimizing materials and interfaces are essential to building such green technologies for decarbonization and sustainability. Particularly, we need unprecedented materials, such as electrocatalysts that can efficiently and steadily produce green chemicals and fuels at scale and generate renewable electricity on demand. Unfortunately, state-of-the-art materials and interfaces—metals and oxides that make hydrogen by splitting water or generate key chemicals for plastics, fertilizers, or pharmaceuticals from CO2 and wastewater—are far from being optimal, exhibiting sluggish reaction kinetics for complicated multi-step processes and fast degradation under realistic, yet harsh operating reaction conditions.
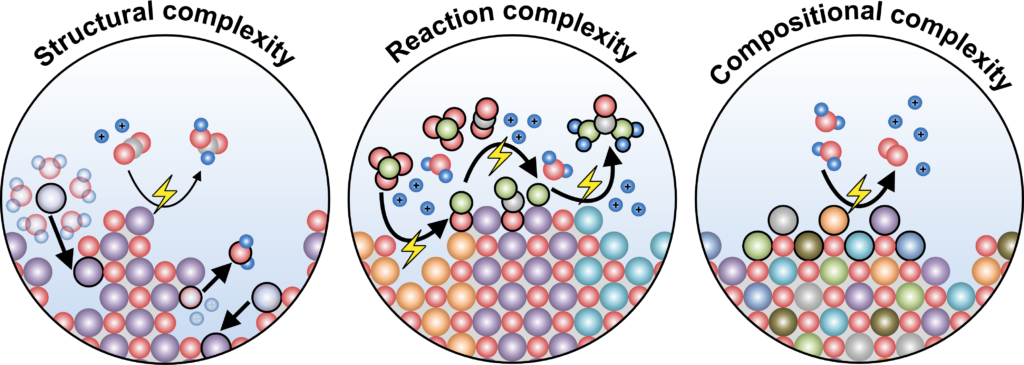
Our Research Questions
Our research group works at the forefront of research in combining materials science with machine learning to identify new physical principles and discover solid-state materials to help revolutionize negative emissions science and renewable energy technologies. We specifically focus on addressing three formidable knowledge gaps that have haunted the understanding and design of materials and interfaces for decarbonization and sustainability for decades:
- Structural complexity: How to unravel the electrochemically-induced surface transformations of materials to unveil the atomic nature of evolved reaction sites?
- Reaction complexity: How to elucidate the intricate interfacial reaction networks to boost the electrification of intricate, underexplored multi-step processes?
- Compositional complexity: How to optimize the compositions and orderings in multinary materials to overcome the trade-off between reactivity vs. durability?