Quantum Dot Heterostructures for Light Harvesting, Excited-State Charge Transfer and Redox Photocatalysis
We use coordination chemistry, organic chemistry, linker-assisted assembly, and successive ionic layer adsorption and reaction to organize nanoparticles into hybrid materials and heterostructures and to attach light-harvesting chromophores to surfaces of nanoparticles and thin films. Our goal is to assemble materials that undergo excited-state charge transfer – separating photogenerated electrons from holes and maximizing lifetimes of these charge carriers – and redox photocatalysis.
We typically use time-resolved spectroscopy to characterize excited-state interfacial charge-transfer processes and photoelectrochemistry and photochemistry to evaluate photocatalytic performance. A central theme of our research is to understand the influence of interfacial composition and electronic structure on the dynamics and yield of excited-state charge-transfer processes and the mechanism and efficiency of redox photocatalysis. Additional details on several current research projects in our group are as follows.
Quantum Dot-MxV2O5 Heterostructures: Exploiting Quantum Confinement and Intercalative Mid-Gap States in Interfacial Charge Transfer and Photocatalysis
As part of a broader collaborative project, we are fabricating quantum dot (QD)-MxV2O5 heterostructures (where M is a main-group or transition metal cation and x is its stoichiometry) with intriguing electronic and optical properties for light-harvesting, charge transfer, and redox photocatalysis. MxV2O5 materials exhibit intercalative mid-gap electronic states that can accept photogenerated holes from QDs on ultrafast time scales. This charge-separation mechanism enables productive redox chemistry, such as the reduction of protons to hydrogen, to outcompete electron-hole recombination. We have now reported on the synthesis, characterization, excited-state charge-transfer reactivity, and photocatalytic performance of a range of heterostructures in which chalcogenide QDs are interfaced with, for example, Pb0.33V2O5, Sn0.23V2O5, and Sb2VO5.
Figure 1: Schematic representation of Sb2VO5 and the energetic offsets and excited-state charge-transfer mechanisms of Sb2VO5/CdS heterostructures that lead to photocatalytic hydrogen evolution. (From Zaheer et al. Chem Catal 2024, 4, 100844. DOI:10.1016/j.checat.2023.100844)
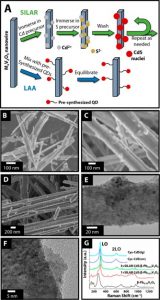
Figure 2: (a) Assembly of QD/MxV2O5 heterostructures via SILAR and LAA, (b-f) electron microscopy images of various heterostructures, and (g) Raman spectra of CdS/b-Pb0.33V2O5 heterostructures prepared via SILAR and LAA. (From Handy et al. Chem. Mater. 2022, 34, 1439. DOI:10.1021/acs.chemmater.1c03762
Recent publications on QD/MxV2O5 heterostructures:
- Zaheer, W.; McGranahan, C. R.; Ayala, J. R.; García-Pedraza, K.; Carrillo, L. J.; Rothfuss, A. R. M.; Wijethunga, U.; Agbeworvi, G.; Giem, A. R.; Andrews, J. L.; Handy, J. V.; Perez-Beltran, S.; Calderon-Oliver, R; Ma, L.; Ehrlich, S. N.; Jaye, C.; Weiland, C.; Fischer, D. A.; Watson, D. F.; Banerjee, S. “Photocatalytic Hydrogen Evolution Mechanisms Mediated by Stereoactive Lone Pairs of Sb2VO5 in Quantum Dot Heterostructures.” Chem Catalysis 2024, 4, DOI:10.1016/j.checat.2023.100844
- Handy, J. V.; Zaheer, W.; Rothfuss, A. R. M.; McGranahan, C. R.; Agbeworvi, G.; Andrews, J. L.; García-Pedraza, K. E.; Ponis, J. D.; Ayala, J. R.; Ding, Y.; Watson, D. F.; Banerjee, S. “Lone but Not Alone: Precise Positioning of Lone Pairs for the Design of Photocatalytic Architectures.” Mater. 2022, 34, 1439-1458. DOI:10.1021/acs.chemmater.1c03762
- Abdel Razek, S.; Popeil, M. R.; Wangoh, L.; Rana, J.; Suwandaratne, N.; Andrews, J. L.; Watson, D. F.; Banerjee, S.; Piper, L. F. J. “Designing Catalysts for Water Splitting Based on Electronic Structure Considerations.” Struct. 2020, 2, 023001. DOI:10.1088/2516-1075/ab7d86
- Chauhan, S.; Sheng, A.; Cho, J.; Abdel Razek, S.; Suwandaratne, N.; Sfeir, M. Y.; Piper, L. F. J.; Banerjee, S.; Watson, D. F. “Type-II Heterostructures of α-V2O5 Nanowires Interfaced with Cadmium Chalcogenide Quantum Dots: Programmable Energetic Offsets, Ultrafast Charge Transfer, and Photocatalytic Hydrogen Evolution.” Chem. Phys. 2019, 151, 224702. DOI: 10.1063/1.5128148
- Cho, J.; Sheng, A.; Suwandaratne, N.; Wangoh, L.; Andrews, J. L.; Zhang, P.; Piper, L. F. J.; Watson, D. F.; Banerjee, S. “The Middle Road Less Taken: Electronic-Structure-Inspired Design of Hybrid Photocatalytic Platforms for Solar Fuel Generation.” Chem. Res. 2019, 52, 645-655 (cover article). DOI:10.1021/acs.accounts.8b00378
- Andrews, J. L.; Cho, J.; Wangoh, L.; Suwandaratne, N.; Sheng, A.; Chauhan, S.; Nieto, K.; Mohr, A.; Kadassery, K. J.; Popeil, M. R.; Thakur, P. K.; Sfeir, M. Y.; Lacy, D. C.; Lee, T.-L.; Zhang, P.; Watson, D. F.; Piper, L. F. J.; Banerjee, S. “Hole Extraction by Design in Photocatalytic Architectures Interfacing CdSe Quantum Dots with Topochemically Stabilized Tin Vanadium Oxide.” Am. Chem. Soc. 2018, 140, 17163-17174 (cover article). DOI:10.1021/jacs.8b09924
Quantum Dot-MoS2 Heterostructures for Light-Harvesting, Charge Transfer, and Redox Photocatalysis
Our collaborative team has recently developed syntheses of QD/MoS2 heterostructures in which chalcogenide QDs are interfaced with MoS2 nanosheets via linker-assisted assembly or successive ionic layer adsorption and reaction (SILAR). Photoexcitation of QDs leads to the transfer of electrons to MoS2, and charge transfer can be exploited in the photocatalytic reduction of protons to hydrogen in the absence of a solvated co-catalyst. Charge-transfer dynamics and photocatalytic performance are tunable with properties of the QD/MoS2 interface
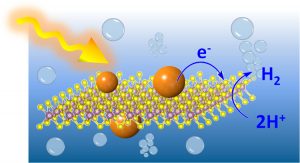
Recent publications on the QD/MoS2 heterostructures:
- Rothfuss, A. R. M.; Ayala, J. R.; Handy, J. V.; McGranahan, C. R.; García-Pedraza, K. E.; Banerjee, S.; Watson, D. F. “Linker-Assisted Assembly of Ligand-Bridged CdS/MoS2 Heterostructures: Tunable Light-Harvesting Properties and Ligand-Dependent Control of Charge-Transfer Dynamics and Photocatalytic Hydrogen Evolution.” ACS Appl. Mater. Interfaces 2023, 15, 39966-39979. DOI:10.1021/acsami.3c06722
- Cho, J.; Suwandaratne, N. S.; Razek, S.; Choi, Y.-H.; Piper, L. F. J.; Watson, D. F.; Banerjee, S. “Elucidating the Mechanistic Origins of Photocatalytic Hydrogen Evolution Mediated by MoS2/CdS Quantum-Dot Heterostructures.” ACS Appl. Mater. Interfaces 2020, 12, 43728-73740. DOI:10.1021/acsami.0c12583
Fabrication of Quantum Dot Heterostructures via Covalent Bonding between Capping Ligands
We tether QDs to each other by forming covalent bonds between terminal functional groups of adsorbed capping ligands. To date, we have focused on the N,N’-dicyclohexylcarbodiimide (DCC)-mediated formation of amide bonds between capping groups of CdS and CdSe QDs to yield QD heterostructures with programmable optical and electronic properties. Additional surface-chemistry steps can enable the formation of bilayers of QDs on charge-accepting semiconductor substrates. Our approach offers two advantages over the use of bifunctional ligands to tether QDs to one another. First, DCC-mediated coupling enables the selective attachment of two different QDs to each other to yield exclusively heterostructures without the undesired formation of homostructures of identical QDs. Second, QDs primed for incorporation into heterostructures have no affinity for each other in the absence of DCC, enabling us to trigger the assembly of materials and to control the rate and extent of coupling reactions and the relative numbers of constituent QDs in molecularly-tethered assemblies. Importantly, we have discovered that our first-generation amide-bridged CdS/CdSe QD heterostructures undergo efficient photoinduced inter-QD charge transfer.
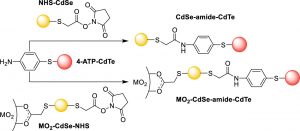
Figure 4: Mechanism of DCC-mediated assembly of CdSe/CdTe QD heterostructures (top) and metal oxide-immobilized heterostructures (bottom). (From McGranahan, C. R. ACS Appl. Mater. Interfaces 2021, 13, 30980-30991. DOI:10.1021/acsami.1c05653)
Recent publications on QD heterostructures and materials assembly
- McGranahan, C. R.; Watson, D. F. “Influence of Donor-to-acceptor Ratio on Excited-state Electron Transfer within Covalently Tethered CdSe/CdTe Quantum Dot Colloidal Heterostructures.” J. Chem. Phys. 2022, 156, 054706. DOI:10.1063/5.0078549
- McGranahan, C. R.; Wolfe II, G. E.; Falca, A.; Watson, D. F. “Excited-State Charge Transfer and Extended Charge Separation within Covalently Tethered Type-II CdSe/CdTe Quantum Dot Heterostructures: Colloidal and Multilayered Systems.” ACS Appl. Mater. Interfaces 2021, 13, 30980-30991. DOI:10.1021/acsami.1c05653