Research
Our research intersects the multidisciplinary fields of thermal science and engineering, renewable energy, nanoengineered materials, novel imaging, and machine learning. We aim to bring about transformational efficiency enhancements in energy (power generation, oil and gas, renewables), water, agriculture, transportation, building, and electronics cooling by fundamentally manipulating heat-fluid-surface interactions across multiple length and time scales.
Nanoengineered Surfaces
Interactions between liquids and solids are ubiquitous in our physical environment and are typically characterized by the wetting angle that a liquid droplet makes on the solid surface. While wettability on flat and homogeneous surfaces has been researched extensively, recent advances in micro-/nanofabrication and coating technologies have enabled the development of smart engineered surfaces. Fundamental understanding of the wetting and liquid propagation behavior on these surfaces is important for a range of applications such as microfluidics, thermal management, lab-on-a-chip devices, desalination, optical, and biological systems.
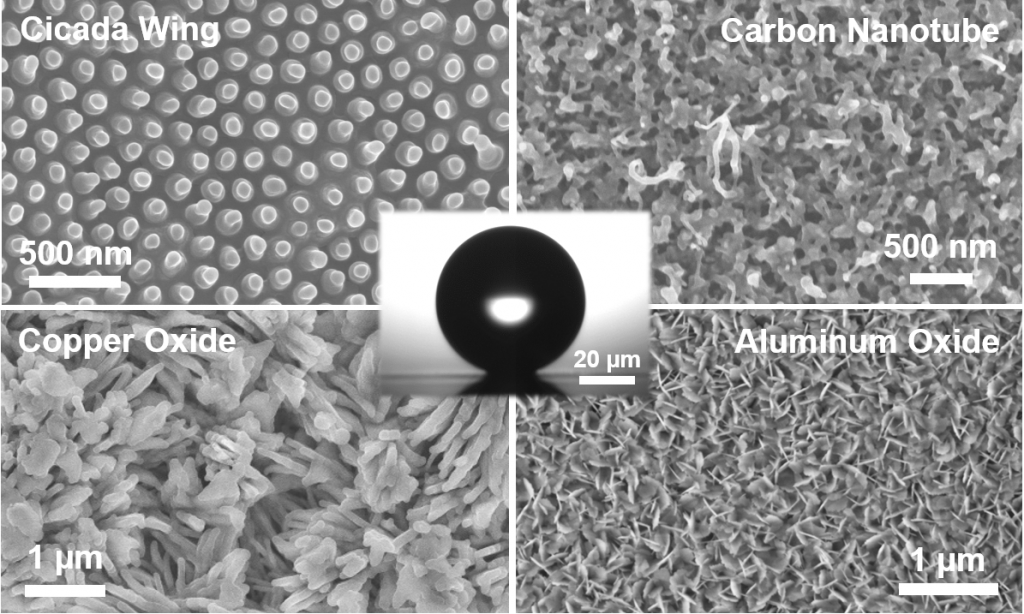
We work towards developing a better understanding of the change in wettability due to surface engineering, chemical heterogeneity, and in the presence of liquid-vapor phase change phenomena. These studies are critical for elucidating the underlying physical mechanisms behind the other research topics being investigated in the lab. For example, hydrophilic surfaces made superhydrophilic due to structuring have resulted in enhancement of boiling and thin-film evaporation heat transfer. Conversely, hydrophobic structured surfaces, i.e. superhydrophobic surfaces have recently shown a promise to push the limits of condensation heat transfer.
Condensation Heat Transfer
Condensation is a phase change phenomenon often encountered in nature, as well as in industry for applications including power generation, thermal management, desalination, and building environmental control. For the past nine decades, researchers have focused on creating surfaces allowing condensed droplets to be easily removed by gravity for enhanced heat transfer performance. Recent advancements in nanofabrication have enabled increased control of surface structuring for the development of superhydrophobic surfaces with even higher droplet mobility and, in some cases, coalescence-induced droplet jumping.

We theoretically, numerically, and experimentally investigate superhydrophobic and oleophobic surfaces for enhanced condensation heat transfer for water, petrochemical fluid, and refrigerant condensation. We seek to develop scalable fabrication techniques for creating superhydrophobic surfaces with experimentally demonstrated heat transfer enhancement. In addition, we focus on studying the fundamental degradation mechanisms of these surfaces with significant collaboration with industry to develop durable and robust coatings that can last for multiple years without re-application.
Machine Learning Driven Design for Boiling Heat Transfer


Boiling is a ubiquitous process in numerous applications, including nuclear power plants, fossil fuel power plants, thermal management, water treatment, and industrial process steam generation. Predicting and enhancing boiling heat transfer performance, however, is notoriously difficult and still largely relies on empirical correlations and experimental observations after over a century of research and development. This is due to the complex nature of boiling that couples fluid dynamics (bubble nucleation, growth, departure) and heat and mass transfer at multiple scales (from nano to macro).
In a collaboration with computer scientists, we develop machine learning (ML)-assisted models to predict and optimize boiling heat transfer. We (i) fabricate and characterize a variety of engineered surfaces, (ii) perform boiling curve acquisition experiments on these surfaces to produce a high-quality training dataset, (iii) design, train, and validate ML models using our experimental data along with comprehensive input variables, such as material and fluid properties, and (iv) use the validated ML models to optimize boiling performance and perform inverse design to determine the associated detailed surface features.
Imaging Dynamic Phase Change Phenomena
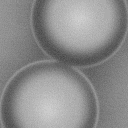
High speed imaging of droplet coalescence on superhydrophobic surface

High speed imaging of droplet jumping on superhydrophobic surface

Droplet microgoniometry
on superhydrophobic surface
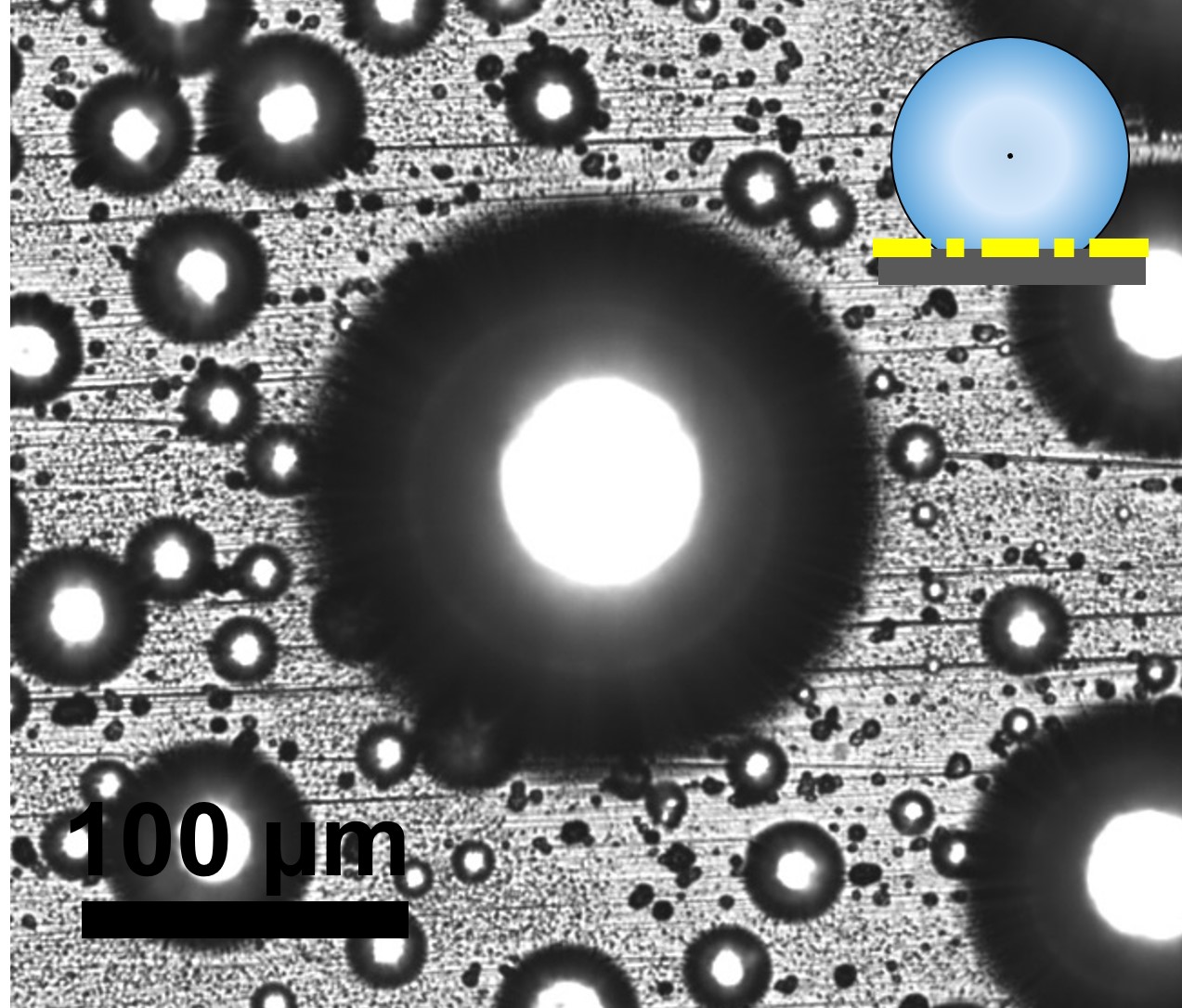
Optical microscopy
on superhydrophobic droplet

Ray optics simulation
on superhydrophobic droplet
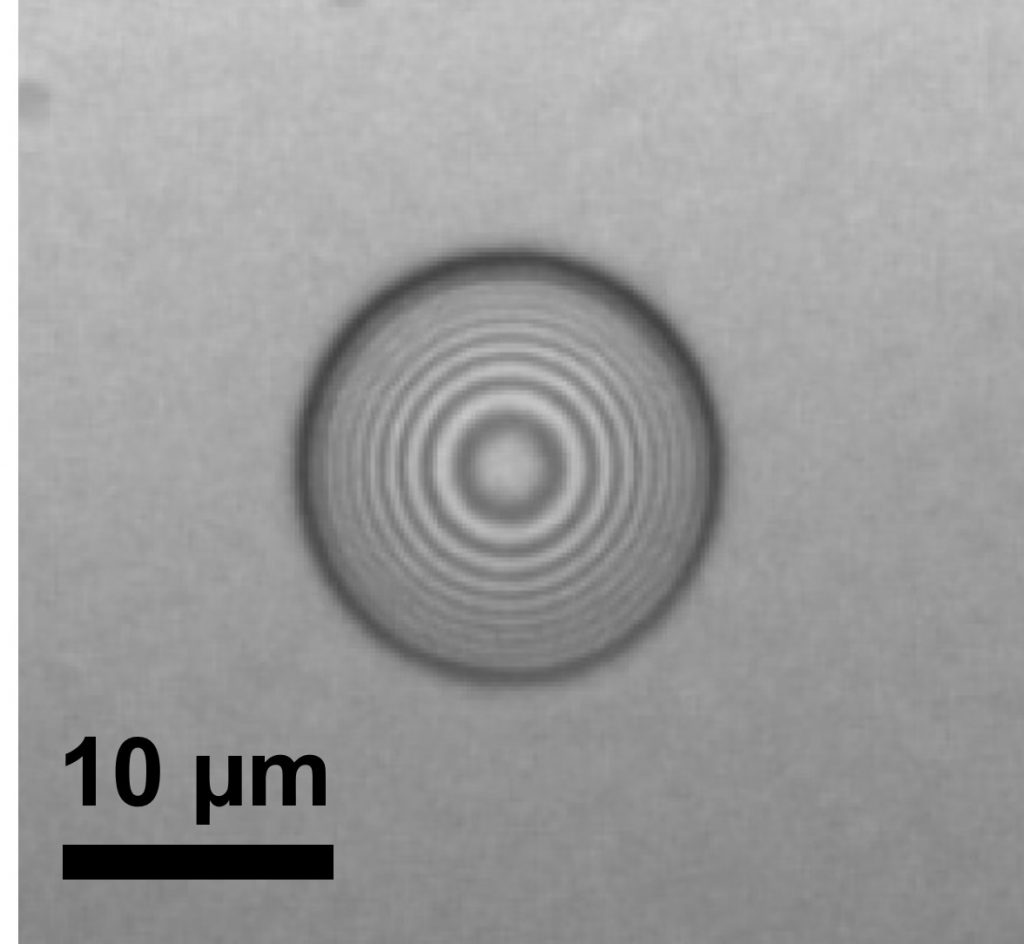
Wave interference
on hydrophilic droplet
The study of micro/nanoscale phase change phenomena as well as wetting interactions between droplets, bulk liquids, and solids is limited by the lack of observation techniques capable of resolving the required spatial and temporal scales. We address these challenges through the simultaneous extraction of mechanical and chemical information using novel sensing platforms developed such as focal plane shift imaging, ray optics simulation, and wave interference. We investigate droplet-droplet and droplet-surface interactions at micrometric length scales with high temporal resolution. The fundamental understanding unlocked form the study of these interaction mechanisms has enabled us us to develop key innovations for many applications and devices.